How Artificial Photosynthesis is the key to a greener future
- Shawn Nassabi
- Jul 15, 2020
- 7 min read
Updated: Jul 20, 2020
By: Minseo Kim, Nyah Lawrence, Arundhati Surendhran, Yomna Mohamed and Tanvi Singh
As the looming crisis of global warming is not getting any smaller, artificial methods have been deemed necessary to slow down or even reverse the effects of greenhouse gases on the atmosphere. Biomimetics, a name coined by Otto Schmitt in the 1950s for the transfer of ideas and analogues from biology to technology, has produced some significant and successful devices and concepts in the past 50 years but is still empirical.
In places where growing trees are an economic or a physical impossibility, biomimetic trees will be extremely helpful to ensure that excess CO2 is being used up.
A biomimetic tree is a technological imitation of a tree which is used to solve problems created by excess carbon dioxide in the atmosphere.
This type of technology uses mechanisms derived from biological processes to use up the greenhouse gas that is a danger to the Earth’s ozone layer which will cause irreparable damage to our surroundings. It is composed of a mechanism using photochemical cells, which will use the artificial photosynthesis process to convert CO2 into more useful things.
The main purpose of such a tree, like any other form of biomimicry, is to increase the efficiency and positive consequences of already existing biological/botanical forms on Earth. The biopolymer material of the tree will ensure that once the photochemical cells have been used and are no longer useful, the tree itself will naturally degrade and in turn become useful to its surroundings, rather than a pollutant. Using a biopolymer will also make sure that the amount of water necessary to sustain these trees will decrease, as that can be an environmental and economical drawback.
Artificial photosynthesis
Artificial photosynthesis is a process that mimics photosynthesis by converting carbon dioxide into carbohydrates or energy/fuel and oxygen using sunlight and water. To do this successfully, you need to be able to split water molecules and harvest sunlight. In plants, this can be done with chlorophyll using proteins and enzymes but to do this artificially you would need catalysts and an artificial method to split water. Harvesting sunlight can be done using solar cells which are relatively easy and accessible, this sunlight can be used as electricity.
The hardest part of this process is the splitting of water. Scientists have run experiments and found that the use of a catalyst such as manganese, cobalt oxide, or titanium oxide can be used to split the water molecules into hydrogen ions, electrons and oxygen. They do this by reorganising the electrons to break bonds and form new ones. The electrons released are essential to reduce carbon dioxide into usable fuels. Cobalt oxide, for example, is an effective catalyst as it can photooxidise water into hydrogen and oxygen. The problem with this, however, is that the efficiency is too low for commercial use.
This process can create liquid hydrogen which can be used as fuel in hydrogen engines. It can also be used in hydrogen fuel cells which would generate electricity by reversing the photosynthesis process. The hydrogen can also be used to creates alkanes and alcohols which can be used to fuel as well.
In artificial forests, this artificial photosynthesis technology will be used on a large scale to convert huge amounts of carbon dioxide and water into hydrogen, oxygen, and alcohols or alkanes.
This means there will be a carbon-neutral method of creating fuels, lowering the amount of carbon dioxide in the atmosphere by a lot since currently, the most common type of fuels are fossil fuels, which serve to release carbon dioxide.
Carbon Capture
For the process of Artificial Photosynthesis to be a success, a source of carbon dioxide is needed, and the most suitable method for capturing CO2 from the air, no matter what its concentration in the atmosphere is, is by adsorption.
Adsorption is the adhesion of molecules, from a gas or a liquid, to a surface. This is due to the molecules’ unbalanced forces that cause them to have residual surface energy, causing them to stick to a solid surface. The adhesion solely depends on the attraction between the unbalanced forces and the chosen solid surface. One can think of adsorption as having a magnet that pulls whatever substance one wants from the air. So, in our case, we want to create a “magnet” that pulls CO2 out of the air.
There are two types of adsorption; physical and chemical. Physical adsorption is the adhesion due to the interaction of intermolecular forces between the gas/liquid molecules and the solid surface’s molecules. Chemical adsorption is the adhesion due to the formation and destruction of chemical bonds. However, these bonds do not create any new substance, they only cause the gas/liquid molecules to “stick” to the solid.
Our ideal solution would be to have a device that attracts the carbon dioxide from the air and attach it to a solid surface without any actual reaction happening and having the ability to detach the CO2 easily and storing it.
A researcher from MIT developed a battery, consisting of electrodes that when charged cause the CO2 from the air to stick to them and when discharged, release the carbon dioxide molecules.
The battery is made up of multiple layers of electrochemical cells consisting of electrodes that are coated with polyanthraquinone-CNT (a carbon nanotube composite) for the cathodes and coated with polyinvylferrocene-CNT for the anodes. The electrochemical cells are layered so that in between them there are passages for the air to pass through to allow for the maximum surface area potential so that as much CO2 as possible is adsorbed.
The polyanthrquinone-coated electrodes capture the CO2 when charged via the carboxylation of reduced quinones.
The batter has an entryway for air so that it passes through layers of the electrode plates and an exit for the air. While the CO2 molecules are stuck the electrode’s surface, the air would be released into the atmosphere and once it’s out, the electrodes are discharged so that the CO2, too, is released. So, for our artificial forest, we would use the released CO2 and turn it into oxygen and useful fuels using artificial photosynthesis.
Catalysts
For every research done by scientists, finding the appropriate catalyst to promote the reaction and efficiency is the true obstacle. Just like there is a myriad of elements all around us, there are also a copious number of catalysts that can be chosen for a reaction.
For the artificial forest, scientists all around the world were successful in finding the appropriate catalyst with sufficient conversion efficiency which is both economical and environmentally friendly in the long run, showing the possibility for the implementation.
According to the researchers from the Tokyo Institute of Technology, they were successful in making a photocatalyst that raises the conversion efficiency of CO2 to CO and HCOOH for about 57%.
This research is very meaningful from the perspective that it is using a cost-effective and widely-available catalyst: Copper and Manganese. Traditionally, we have been using expensive and rare metals like platinum, Ruthenium, Palladium, etc as a catalyst for such research, and this incurred significant costs that were very uneconomical in the long run. However, after this finding, many things can change, and possibly the odds of this to be implemented in the future.
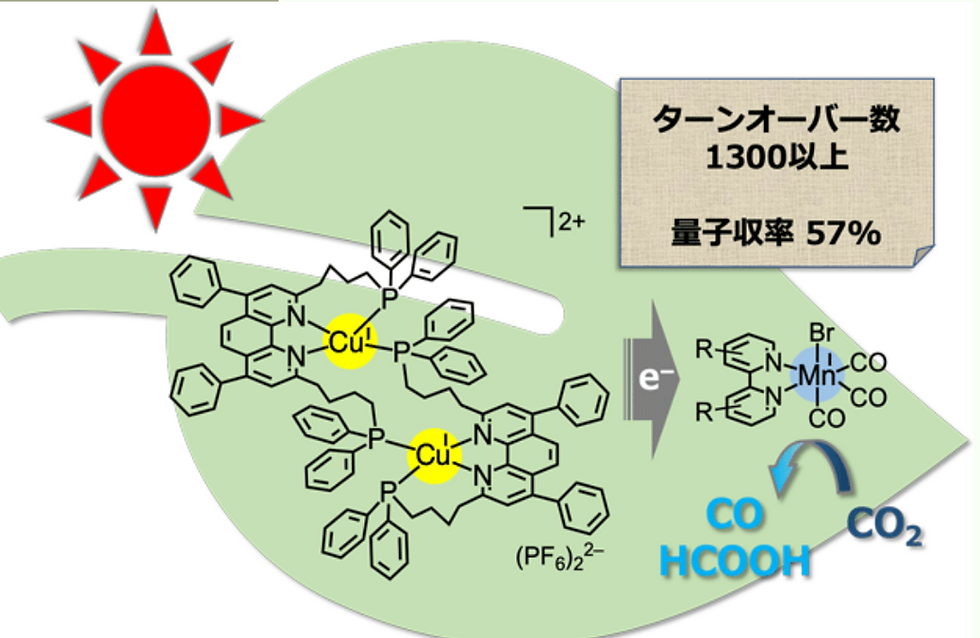
These catalysts can be designed in the form of a tree, just like how researchers in Berkeley Lab have designed, to maximize the output and act just like a tree in an ecological system, absorbing sunlight and carbon dioxide and converting them into oxygen. One special thing about these catalysts is that they can also absorb various forms of light, including UV rays, visible light, etc to provide energy for this Artificial Forest system. Further referencing Berkeley Lab research, the process carried out by such catalysts were:
Harvesting solar energy, generating reducing equivalents, reducing CO2 to biosynthetic intermediates, and then producing value-added materials. These value-added materials like formic acid, hydrogen, oxygen, and fuels could be used to take one great step to reduce carbon dioxide emissions and produce more oxygen supplies on Earth.
Formic Acid, HCOOH, which is converted from CO2, takes a very important role in carrying out the whole process of artificial forest. First, they have a special characteristic that is to save and store hydrogen produced from water splitting very effectively. This means that it would definitely play a crucial role in storing the hydrogen ions produced from the process and later be used for better purposes. In addition, they can react further and be converted into useful fuels which can also be stored and be used later. This acid takes a vital role in artificial photosynthesis, a similar concept to the artificial forest, as it helps it to convert into useful and eco-friendly fuels like methanols, ethanols, and propanols. From this perspective, it can take an important role in storing hydrogen ions, which are expensive to produce and store, and producing useful fuels, which can change the possible ways we obtain fuels in the near future, giving reasons why artificial forests must be implemented.
Photo-biological Methods of Biofuel Production
Another method pursued in regards to ‘artificially’ photosynthesising and reducing carbon emissions is by the usage of genetically modified microorganisms. The research conducted within this field has proven largely successful, with the main issue coming out to be the efficiency of the system. There are multiple studies on how exactly photosynthetic microorganisms can be best utilised for the purpose of producing biofuels while also providing a solution to the increasingly worrying threat of climate change, they include, but are not limited to; the production of hydrogen through nitrogen-fixing bacteria, the production of 1-butanol from GM cyanobacteria, and more.
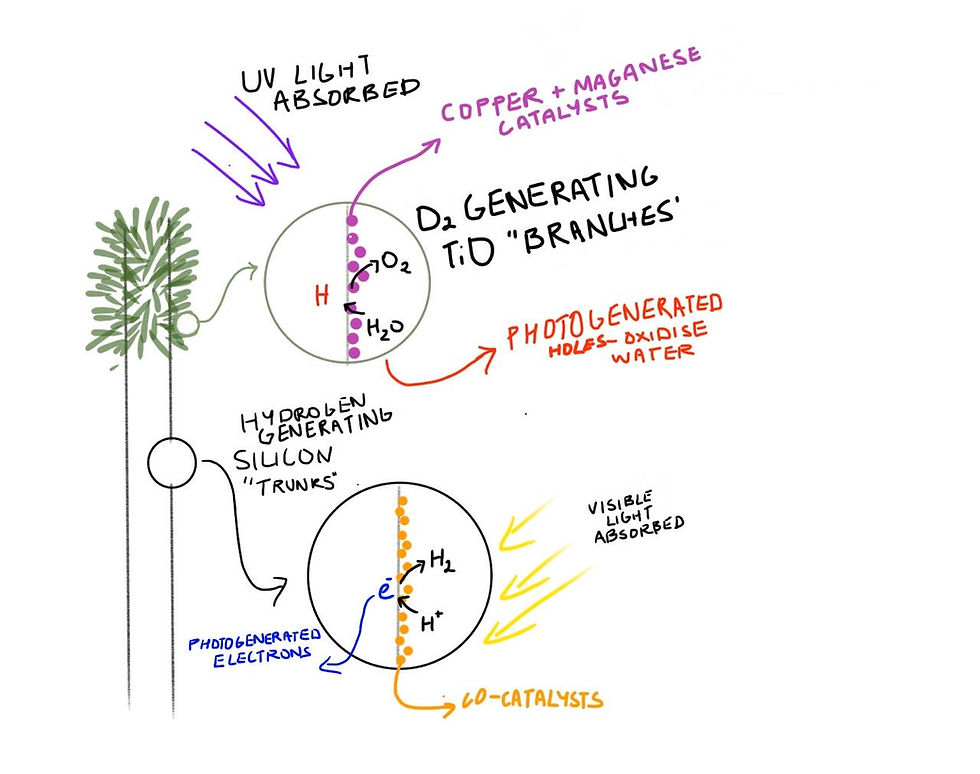
The process by which hydrogen is mainly obtained from photosynthetic microorganisms is primarily through nitrogen-fixing bacteria. They contain nitrogenase, an enzyme that is responsible for the conversion of gaseous or atmospheric N₂ into Ammonia. Hydrogen is the byproduct of this reaction, but is most often not released, and is taken up by hydrogenase, another enzyme. The solution to this is to then erase all hydrogenase activity, and when done so by using insertional mutagenesis, the production of hydrogen was observed.
Obtaining heavier fuels that contain carbon has also been proven possible, with mutant cyanobacteria producing butanol. But the issue we come to see in both these methods is the low efficiency.
Researchers’ primary target is to increase the efficiency at which these processes work, which has furthered the field into an age of not just modifying microorganisms but engineering it.
This concept chiefly works intending to produce bacteria with ‘solar panels’, titled ‘Cyborg Bacteria’. The researchers within the project repurpose the natural defence of some bacteria against mercury, cadmium or lead, whose properties turn these metals into sulphide ions that then exist on the surface of the bacteria, essentially acting as tiny semiconductors. These ‘cyborg bacteria’ produce acetic acid from the basic requirements of photosynthesis, Carbon Dioxide, Water, and Light; boasting an 80% efficiency, six times that of chlorophyll, and four times that of standard solar panels.
However, what we can see from all this research is that humanity has progressed far beyond what we expect, and that hope for the future dwells within scientific pursuit. The reduction of Carbon Emissions is no longer a chore, but something that can produce valuable products that will help fuel our ventures into the distant future, and at the centre of it all, as always, remains the sun.
Reference sites:
Comentários